The principal challenge of this century, in my view, will be adapting to a life without abundant, cheap fossil fuels. It has been the lifeblood of our society, and turns out to have some really fantastic qualities. The jury is still out as to whether we will develop suitable/affordable replacements. But additional challenges loom in parallel. Water is very likely to be one of them, which is especially pertinent in my region. For true believers in the universality of substitution, let me suggest two things. First, come to terms with the finite compactness of the periodic table. Second, try substituting delicious H2O with H2O2. It has an extra oxygen atom, and we all know that oxygen is a vital requisite for life, so our new product will be super-easy to market. Never-mind the hydrogen peroxide taste, and the death that will surely visit anyone foolish enough to adopt this substitution. Sometimes we’re just stuck without substitutes.
Substitution silliness aside, water and energy are intimately related in what has been termed the Energy-Water Nexus (see for example the article by Michael Webber from this conference compilation; sorry about the paywall). We’ll explore aspects of this connection here, touching on pumping water, use of water for the production and extraction of energy, and desalination. As glaciers and snowpack melt and drought becomes more common in the face of climate change, our water practices will need to be modified, hitting energy right in the nexus.
Household Water
Let’s start at the familiar level. A typical San Diego residence uses 14 hundred cubic feet (1 hcf = 748 U.S. gallons = 2831 L) of water each month—working out to 138 gal/day (520 L/day) per person, assuming an average of 2.5 people per residence. Based on backpacking experience, this is more than one hundred times as much water as is necessary to satisfy basic needs.
Note: only 60% of Do the Math readers are from the U.S. The volumetric units in this post will likely elicit a groan or two overseas. Some additional conversions: 1 gallon is 3.785 L; one cubic meter is 264 gallons; and 1 hcf is 2.83 m³.
My own household (two people) averages 20 hcf per year. This past year is slightly anomalous in that we planted a large section of our yard with drought-tolerant California natives, and have supplied 4.25 hcf of water (5.5 inches or 14 cm of rain equivalent) to help establish them in their first year—yes, I record sprinkler use. Even so, our water use over the last year comes to 23 hcf—working out to 47 gal/day (or 38 gal/day ignoring temporary irrigation).
Okay, so I’m shocked² by these sets of numbers. First, I am shocked that we use as many as 38 gallons per day within our house. On what?! We are sparing with showers, each requiring only five gallons and on a roughly two-day cadence. Our low-volume toilets (one of which has a dual flush capability) average something like 1.5 gal per flush, and we don’t flush after every use (of certain types, if you know what I mean). Dishes? Sparing, efficient. Laundry? The same. Vegetable garden? Rainwater catchment (600 gal storage). So it’s hard to get it to add up—yet it must, and I accept that.
The second power of shock (thus the square) is the nearly 10× greater usage (344 gal/day) by typical area households. Yet it’s not an unfamiliar mismatch—also appearing in our use of electricity, natural gas, etc. But where is all this water going?
Presumably, much of it goes into creating green lawns in a semi-arid desert: San Diego typically gets about 10.3 inches, or 26 cm, of rain per year. If I guess that a typical house in San Diego has about 2000 ft² (185 m²) of lawn, then tripling the natural rainfall amount will require 34 hcf of water, or about an extra 3 hcf per month. Hmmm. Not as big a chunk of the monthly 14 hcf as I thought it would be. Maybe I underestimate how much irrigation people are doing. Overwatering, overspray, evaporation, and leaky sprinkler systems may be a big part of the story, but I am still left a bit puzzled. I guess frequent long showers (also energy-intensive), washing clothes maniacally often, flushing every use, hosing off the driveway, washing cars, etc. may all add up.
One final comment on domestic water. Each month, I pay a $19.33 base rate for water service (not including wastewater service, which is a comparable charge). Then it’s $3.61 per hcf of water used. So my monthly water bill comes to about $26. Meanwhile, the average residence pays $70 for their 14 hcf allocation. I’m paying about 2.7 times as much per gallon as the average resident: my reward for conserving, apparently. A further amusement is that my water service issued a “20 gallon challenge,” which asks residents to shed 20 gallons per day per person. Setting aside the temporary native-plant irrigation, our 38 gal/day household usage would mean that rising to the challenge would result in our complete abstention of utility water usage. In fact, it would require each of us to cough up (not literally) a gallon of water each month to donate to the utility. Good thing we have rain catchment.
National and Statewide Usage
The USGS provides national water use estimates every five years. For 2005, the total 410 billion gallons per day works out to about 1330 gal/day per person. This drops in half if excluding water used for cooling power plants. For California, 81% of the withdrawals were for power plants and irrigation, leaving 240 gallons per day per individual in the state.
Energy for Water
A 2006 report pegs the total amount of water-related energy use in California at 52 billion kilowatt-hours annually. This computes to about 6 GW of continuous power expenditure, or 160 W per person. Not a trivial amount. Of the 52 TWh, 32 are performed by the end user for heating, cooling, moving, filtering, or pressurizing the water. The other 20 TWh goes into pumping water across the state, including over mountain ranges. That’s about 8% of California’s electricity demand!
As a lark, if we dumped all 52 TWh of energy into the annual California water supply (40 million acre-feet: just listen to the Europeans howl), how much would the water warm up? Answer: about 1°C. Not all that much. But it illustrates the degree (ha!) to which water heated by 40°C for a shower or by 80°C for cooking pasta is high-value water, relative to the rest.
Combining the energy and volume numbers in another way, each gallon of water in California comes with an average energy price tag of 0.004 kWh, or 0.0015 kWh for the pumped-delivery charge alone. This means that a 1 hcf unit costs just a bit over 1 kWh to deliver.
Water for Energy
But there is another direction to consider as well. Power plants generally use water as a conveyance for waste heat, and withdraw far more water than any other entity in the U.S. (including agriculture). According to the EPA, every kWh of energy delivered demands the participation of 25 gallons (95 L) of water. Most of this is either returned to the source (warmer by 17°C, I calculate) or preserved in a closed system. But 2 gallons are lost for each kWh of energy delivered (regionally variable: much higher in arid regions). Luckily, the resulting kWh is sufficient to deliver another 750 gal to the door, so there’s no spiraling trap preventing us from consuming water in this way.
Obviously, hydroelectricity is another place where water and energy collide. In drought conditions, municipalities may increasingly face decisions about whether to use the contents of their reservoir for water or electricity. Interestingly, according to Webber, each kWh of hydroelectric energy produced results in the loss of 18 gallons of water due to increased evaporation off of manmade reservoirs—over-and-above what would have happened in the natural run of the river. So hydroelectricity is more consumptive than the thermo-electric plants by a fair margin.
Production and processing of our fossil energy commodities also require the use of water. Gasoline consumes a few times its volume in water for production. But from an energy standpoint, at 36.6 kWh/gal, every kWh of energy available in the gasoline takes a small fraction of a gallon of water to produce. Thus electric cars turn out to be more water-intensive than gasoline cars, for instance, if each kWh of electricity production gulps a few gallons of water. For tar sands, the ratio is about 5:1 water:oil-product, plus more for refining. A similar number accompanies oil shale extraction, but in the U.S. the oil shale is primarily situated in arid regions where water availability is limited.
Hydraulic Fracturing (“fracking”) for natural gas appears to require 10 L/GJ of water consumption. For the energy equivalent of one gallon of gasoline, this works out to just about one liter—so not as intensive as tar sands or oil shale, or even gasoline. Mostly this is because gas is happy to flow on its own with little help from water: the water only has to create the cracks (fracks?). However, water does enter the equation in other ways for fracking. Contamination of ground water is a principal concern, and some residents have complained of being able to ignite the effluent from their kitchen sink faucets!
But the real water hog for energy purposes is biofuels. Each gallon of fuel produced may consume something on the order of 1000 gallons of water (regionally variable). That’s about 25 gallons per kWh, to put it in the same units as we discussed for power plants. Naturally, the impact depends on the degree to which irrigation is employed vs. natural rainfall. I would assume that the amount of water used in the refining/processing is similar to that for gasoline and therefore does not contribute much additional burden.
Desalination
Another domain in which energy and water concerns collide is in desalination operations. One possible solution to Southern California’s reliance on water pumped over mountains from the north is to make use of the ocean of water breaking onto the local beaches.
A recent blurb in the NYT announced plans for a desalination facility just north of San Diego that would produce 50 million gallons of water per day—aiming to supply 7% of regional demand by 2020. The price tag is roughly a billion dollars. The conversion factor is thus about $20 for every gallon-per-day of capacity (at large-scale; likely fails at the household level). The estimated cost comes to about $4.80 per hcf, which is higher than the current price of water to the end-user in San Diego. But I’m not knocking it on these grounds: many of our future options will likely be more expensive than the magic carpet ride we’ve enjoyed on the foundation of fossil fuels.
Energetically, the most straightforward approach to desalination is evaporating water and collecting the condensed vapor. Put salt water (or anything moist) in a dark container with clear plastic or glass across the top and place it in the sun. The interior will heat up and evaporate water, which then condenses on the cooler plastic/glass cover. An appropriate sloped-roof geometry allows drip-collection of the water.
Every gram (mL) of water vapor that escapes its liquid birthplace exacts a toll of 2257 J. This is a bit steep. Heating that same parent gram of water by 1°C only costs 4.18 J—which is why a pot of boiling water takes ages to boil away to nothing (see post on boiling water efficiency). A brute force approach like this would demand 2.4 kWh of thermal energy for every gallon of water produced (or 633 kWh/m³)!
Until I did the math, I dreamed of flowing ocean water through a system of shallow, covered trenches, generating fresh evaporated condensate. Low-tech, solar-driven, lovely! We might optimistically capture 50% of the incoming solar energy in the trough-collector, so that each square meter receiving about 5 kWh/day of incident energy could result in one gallon of water. Producing 10% of California’s demand would then require an area 60 km on a side, or a strip of land along California’s entire coastline about 2.5 km wide. Let’s call that infeasible. Darn.
But there are back doors. For one, waste heat from power plants (including nuclear) can be used as the source of energy in a form of co-generation. Also, water can be made to boil vigorously at room temperature in a mostly evacuated system once the pressure drops to about one-fortieth of an atmosphere (~20 Torr). In this scheme, it would take about 34 kWh to pump out 1000 L (1 m³) of water molecules against this pressure differential: a bargain compared to the 633 kWh/m³ from direct heating.
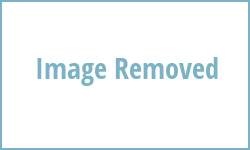
Source: Wikipedia
Most desalination plants use the multi-stage flash distillation process, which employs low-pressure chambers and recovers much of the heat of vaporization as the vapor condenses on the feedwater intake pipes, reducing the amount of direct heating required. These devices tend to achieve about 18–25 kWh/m³, which, again, is a bargain compared to direct heating. Finally, reverse osmosis (RO) is another option, forcing water through membranes that exclude the saline ions. Typical RO installations achieve about 5–7 kWh/m³ (see, for instance, here or here). But the osmosis approach requires high-grade electricity, which if produced in the traditional manner requires about 17 kWh of thermal energy input per cubic meter of water produced. So really these prevalent techniques are not tremendously different energetically, although perhaps the osmosis approach is more finicky in terms of water preparation/filtering, gunked up membranes, etc. RO wins out energetically if the electricity is from non-thermal sources (wind, solar, hydro).
Okay, so now that we have an idea about how desalination is really done, what would it mean from an energetic standpoint if California tried to fulfill a substantial fraction of its water demand from desalination? I’ll use the approximate value of 20 kWh/m³ (thermal) hereafter.
Let’s start with San Diego’s effort to replace 7% of water demand in its 50 MGPD plant. This works out to 160 MW of thermal power. For scale, the San Diego region uses electricity at an average rate of 2.3 GW. So we’re talking a noticeable amount. Extrapolating to 100% desalination for San Diego, we get to 2.3 GW thermal, which would substantially increase local power generation demand. Economically, about half of the negotiated $4.80/hcf cost is in energy. It works out to 15 years to recoup the construction cost for the plant under the (poor) assumption of constant prices.
What about California as a whole? San Diego county is not a heavy agricultural center, so the problem will get harder if trying to satisfy the state’s needs (and therefore the country’s food needs given the national-scale importance of California’s agriculture). California uses 46 billion gallons of water per day. Supplying 25% of this via desalination would require 36 GW of thermal-equivalent power. California runs on 30 GW of electricity, and a total energy budget of 262 GW (thermal; from oil, gas, coal, hydro, nuclear, etc.—according to the EIA). That’s a substantial amount for 25% of our water needs.
Another way to slice this problem is to ask what fraction of California’s water could be provided by using the amount of energy that currently goes into pumping water around the state. We use 20 billion kWh of annual electricity for pumping, translating to about 6.5 GW of continuous thermal power. This amount of thermal power could meet 4.5% of California’s water needs via desalination. When we currently spend 8% of our electricity delivering 100% of our water, and would only meet 4.5% of our water needs by the same energy investment directed to desalination, we can appreciate the crunch.
Will the Nexus Vex Us?
What a surprise—the world is a complicated place with interdependencies. Is the water-energy connection more than an academically interesting tangle? I think so. Water is undeniably important to our physical survival, and energy is the main physical ingredient in our development of modern society. Shortages in either could have major impacts, and their entanglement means that a shortage in one could trigger a shortage in both. Seems like a problem—especially in light of increasing population pressure and intensifying effects of climate change.
Of course my “solution” is frequently to ask why we need as much energy or water or you-name-it-resource as it seems that we do. The fact that I live a perfectly functional life using less than 20% as much electricity, gas, and water as my San Diego cohort sure seems to suggest a viable path away from the crunch.
The good news in all of this is that when faced with difficult limitations, economic factors will assert themselves via the beloved mechanism of skyrocketing prices. People will naturally react by cutting back significantly, as my experience indicates is clearly possible. I am confident that San Diego could still function on a drastically reduced water budget, if needed. Every 60 ft² of roof area in San Diego collects enough rain to provide a gallon per day averaged over the year (1.4 m² collects 1 L/day). So thirst shouldn’t be a problem, even for a few million people. I’m not so optimistic about the odds of grass and ornamental plants surviving serious cutbacks. So while survival is not at stake, our accustomed ways of life may well be endangered.
The bad news is that we appear to be incapable as a society of reacting to a looming situation before economic forces drive us to change. By that time, we have often lost precious years to prepare for a new reality. We tend to want proof that something is a problem before we alter course. Not the smartest of approaches, in my book. Ironically, the political “conservatives” tend to be the most resistant to conserving resources or approaching the future with a conservative, low risk mindset. Growth trumps caution. A key philosophical difference may lie in one’s sense of whether growth solves problems (debt, hunger, unemployment), or creates them. The answer does not have to be static—especially in a world of finite resources transitioning from the “empty” to the “full” state. We may well see an evolution from a world in which “growth the solution” more and more is perceived as “growth the problem.” I think attitudes are already shifting in this direction.